Project: Microfluidic Simulation
In the context of microfluidic simulation, the finite element modeling tool COMSOL is used to describe complex systems and understand their dynamics. It is applied to:- a microfluidic diode for controlled drug delivery:Micropumps are used to automatically and safely inject drugs to the patient (insulin in the case of Diabetes). Typically, micro-pumps have a valve that deteriorates over time. Therefore, a new generation of micro-pumps uses a pumping chamber with a diaphragm that allows the liquid to be pumped. The crucial point is to control the flow of drug to the patient while avoiding the flow of physiological fluid to the micro-pump.We then study an original structure of the microfluidic channel connecting the inlet and outlet of the micro-pump (Figure 1). The velocity field of the fluid is presented on figure 1: the difference of the flows in the opposite directions allows an operation close to that of a diode in electronics with "on" and "off" directions. This is why we call it a microfluidic diode. For its integration in the micro-pump, the absence of removable valves allows a fast and inexpensive manufacturing (by 3D printing for example).
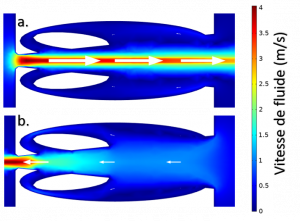
This study is the subject of a collaboration with the Biomedical Engineering Laboratory of Tlemcen (Algeria).
- A concentration gradient microfluidic device for the study of chemotaxis: It has been shown that immune cells have a mode of movement without adhesion but controlled by the gradient of chemokines present in the solution. This gradient can be controlled via flow control in the device. For these studies, it is therefore necessary to design a device with a stable chemokine gradient in time and with a complete control of the flows in the different compartments of the device. The modeling of chemokine diffusion with time was performed in the whole device. The simulation results compared with the experimental results confirmed the domination of the diffusion phenomena on those of convection in the creation of the chemokine gradient in the device (figure 2).
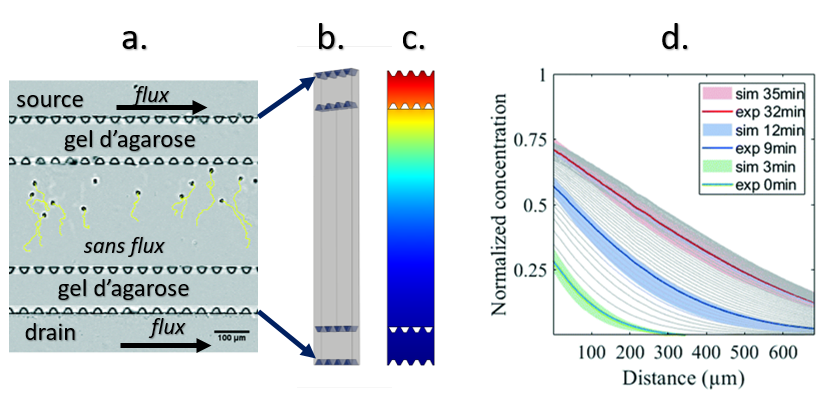
This study is the subject of a collaboration with the Inflammation and Adhesion Laboratory (LAI) (Marseille).
- the study of thrombosis : Venous thromboembolic disease is the 3rd leading cause of cardiovascular death in developed countries. Venous thrombosis occurs when a blood clot forms in a vein. It most often affects the lower limbs and settles in a deep vein. It is then called Deep Vein Thrombosis (DVT). This clot can break off and obstruct a pulmonary artery, forming a pulmonary embolism. The mechanisms of DVT are still poorly understood. The physiopathology of venous thromboembolism is complex and multifactorial. In this project we are interested in the links between different factors such as venous valve stiffness and genetic risks on thrombus formation. We started our study by simulating neutrophil deposition in a microfluidic device representing the deep vein (Blood 137:2256-2266 (2021)).
This study is a collaboration with the Center for Cardiovascular and Nutrition Research (C2VN)
- understanding the contraction of a drop containing molecules of interest on a surface or in a microfluidic flow: (projects: Biomimetic Microparticles and Nucleation in confined environments).
The contraction of sessile drops may seem very simple to describe but in reality several physical phenomena are in competition: for example evaporation, diffusion, phase change, convection and the Marangoni effect (fig. 3). The model becomes more complicated when an ensemble of communicating drops is studied. The understanding of the contraction of small volume sessile drops (from picoliter to femtoliter) will open doors to a wide range of applications: for the understanding of nucleation and crystallization of molecules of pharmaceutical interest (for example) or in the field of medical devices (DNA chips, diagnostics by analyzing the deposition patterns of biofluid drops). The strength of our approach lies in the convergence between modeling and the experimental platform.
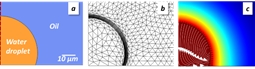
Non-permanent staff involved in the projects:
2017-2018 Ahmed Slami, Doctorant au laboratoire Génie Biomédical de Tlemcen (Algérie)
2019 Maxime Imbert, Stage M1 Bio-ingénierie des Tissus et des Implants–Aix-Marseille Université
2017 Duglas Dunga, Mobilité d’études en 4ème année Génie Biomédical - Polytech Marseille